Intracranial and Extracranial Collateral Circulation
The topic of collateral circulation comes up often in evaluation of intracranial vascular disease, particularly stroke. While a myriad collateral pathways is concievable, some venues are more commonly encountered and therefore deserve praticular attention. Presence of robust collaterals may mitigate effects of occlusion, whereas poor collateral support can result in a devastating stroke. The extent of collateral support depends on genetic factors, both macro and microscopic (some people just have better ones), chronicity of vascular condition in question (collaterals can enlarge over time signficantly) and, most significantly, on physiologic parameters such as perfusion pressure and capacity for autoregulatory dilatation.
The latter factor can not be possibly under-emphasized. According to Medical Dictionary, the definition of collateral circulation is “that carried on through secondary channels after obstruction of the principal channel supplying the part”. http://medical-dictionary.thefreedictionary.com/collateral+circulation (I would consult Wikipedia, but today is Wednesday, January 18th, 2012, and Wikipedia is down for the day). The definition, which I think is very much on target, implies that the concept of collateral circulation is only valid as a response to pathology. It is best to remember that collateral circulation is a pathophysiologic phenomenon — with the implication that physiology represents and integral part of the collateral solution. This physiologic component, I think, tends to be relatively neglected, while the anatomical portion is disproportionately over-emphasized. In this way, the study of “collateral pathways” — the myriad ways in which more than one artery can supply the same territory — is carried ad nauseum, while their dependence on physiologic parameters is sadly overlooked.
This deficiency, i think, has become more prevalent with the success of cross-sectional imaging, which at this point in time remains, overwhelmingly, a static imaging approach. I hope that this will change, as both CT and MR continue gaining dynamic dimention, from perfusion techniques to multipass CT angiography (which, I believe, is a crime against double-stranded DNA) to time-resolved MR sequences such as TWIST and TRICKS. As angiographers, I believe, we occupy superior position from which the dynamic component of collateral circulation can be evaluated. It is also clear that, while anatomical collateral pathways can be appreciated with relative ease, the significance and extent of physiologic factors are much more difficult to measure, while holding equal importance. The examples are many — a given collateral pathway may be sufficient or insufficient depending on its perfusion pressure. When we say that someone is young, and therefore likely has good collaterals, what does that mean? Is it presence of robust anatomical pathways or, more importantly, their capacity for autoregulatory adjustment (dilatation), which makes all the difference on the leptomeningeal level.
One way of addressing both anatomical and physiologic components of collaterals is by considering 1) Presence (anatomical) and 2) “Robustness” (physiological) as separate variables. To this end, the situation can be thought of as a analogous to basic laws of electricity. I=V/R. Voltage is perfusion pressure, R is vascular bed resistance (analogous to autoregulatory adaptation), and I (current) is the sum total of available anatomical pathways. Those of us still familiar with the concept will be able to draw their own conclusions. I hope that, time permitting, this section will be able to do fuller justice to the physiologic component of the collateral concept, so that the following long discussion of anatomical pathways is not taken as proof of deafness to my own better judgement.
Anatomic Collaterals
In terms of organizing one’s thoughts about anatomic collaterals, I find it helpful to consider the subject in “distal to proximal” terms. Starting from most distal — i.e. capillary level, and falling back to branch and ultimately back to great vessels, with proportionate increase in number of collateral pathways provides one type of framework. Finally, a discussion of collaterals is a great way of learning neuroanatomy, both in its “theoretical” and “applied” forms.
This concept is helpful because collaterals are always present to some extent. The question is whether they are adequate for the situation at hand — a physiologic consideration. The difficult problem is how to judge what will be “adequate”. Noninvasively, this is best exemplified by the diffusion-perfusion mismatch. Essentially this is a black box approach — regardless of what collaterals there are, the method estimates whether these support systems are adequate, for the moment, to keep brain alive. Angiographically, both individual collateral routes as well as their functional effectiveness can be evaluated. The latter can be guestimated based on the amount of angiograhic delay between the normal and at risk territories. We consider a delay of 1 second or less to be evidence of excellent collateral support. For example, we do balloon test occlusions under anasthesia, and use the above 1 second rule to determine if the patient passes or fails the test. This turns out to be as good or better than clinical testing of awake patients. Again, this is our protocol, and there are many others.
Distal collateral pathways
We begin with consideration of collaterals at the capillary bed. On a microscopic level, the capillary bed is a network of interconnected vessels. In practice, these connections are not adequate to provide meaningful circulatory support beyond the level of the capillary. What happens when a submillimeter vessel is occluded? The all-too-familiar UBO’s of the elderly patient’s brain MRI, widely considered to be “sequela of microvascular disease”, most likely represent small infractions in the territory or the occluded submillimeter vessel. The apperance is very nonspecfic and similar T2 hyperintensity can be seen in myriad other diseases. However in the “appropriate clinical scenario”, there is strong evidence to point to microvascular etiology.
Segmental level collaterals distal to the circle of Willis — leptomeningeal (convexity) and other
When a 1 mm or so branch vessel is occluded, typically over the brain convexity, collateral support, when available, takes form of retrograde flow through other convexity branches. For example, a high convexity MCA branch may be rescued by an anterior cerebral artery sufrace vessel, also known as leptomeningeal collateral support. The success of these critical pathways depends, to large exent, on physiologic factors such as autoregulatory dilatation; while individuals differ widely in their capacity for autoregulatory support, it is fair to say that, for a given individual, this capacity diminishes with age. This kind of leptomeningeal support is at the heart of diffusion/perfusion mismatch analysis. When adequate flow through leptomeningeal channels is maintained, a larger “penumbra” (region of blood-starved but still viable brain) may be sustained in face of large proximal vessel occlusion, such as the M1 segment. The area of the brain in danger, exemplified by Time to Peak, Mean Transit Time, or classically Cerebral Blood Flow measurements, is compared with the measure of Cerebral Blood Volume, corresponding roughly to the region of “dead brain.” A mismatch exists when the region of reduced CBV is significantly smaller than CBF, MTT, or TTP, implying that a portion of the brain is experiencing reduced blood flow but not yet brain infarction. Thus, a perfusion scan is an indirect measure of extent of collateral support. As such, it may be more sensitive to infarction than visualization of collateral pathways on cerebral angiography. Of course, the extent of penumbra and “core” is influenced by physiologic factors, such as perfusion pressure and vascular resistance.
An example below illustrates this concept, whereby A2 segment occlusion is associated with prominent but delayed reconstituion of ACA territory by ipsilateral MCA convexity branches. However, perfusion CT shows no evidence of mismatch — the entire ACA territory is infarcted by CT criteria. In the end, the territory in question shows infarction on a conventional CT. As such, functional value of collateral support may be roughly quantified during angiography by the lengh of time it takes for the collateral network to catch up to the “normal” phase. For example, if the normal brain in already in the venous phase, but the circulatory bed in need of collateral support is still in the arterial phase, the support is inadequate. As a rule of thumb, a 1 second lag time (two frames in a 2 frame/sec aquisition) is considered as a “pass.” The same rule applies during balloon test occlusions.
Patient 1 — inadequate collaterals in case of A2 occlusion.
CT Perfusion demonstrating no evidence of penumbra.
Catheter angiogram of the same patient immediately following CT perfusion, demonstrating MCA collateral vessels backfilling ACA territory in this patient with proximal left A2 occlison. There is no parenchymal blush in the ACA terrritory however and the collaterals are very late in their apperance in comparison with the MCA. The patient remained hemiplegic.
Patient 2: Convexity ACA-MCA collaterals: adequate collateral support in case of M1 occlusion.
This patient underwent CT perfusion study in setting of acute aphasia and hemiparesis. A prominent mismatch is present on CT perfusion with delay in time to peak but relatively preserved rCBV, with exception of basal ganglia (M1 thrombus will nearly always take out the lenticulostriate vessels which are effectively end-arteries without signficant collaterals)
Angiographic study (below) shows robust convexity collaterals reconstituting MCA territory, to a much better extent than seen for patient 1. Collateral support is provided via conexity ACA and temporal lobe PCA (not shown) collaterals.
Post-thrombolysis CT (below) shows recanalization of the MCA territory. Luxury perfusion is seen in the dead basal ganglia, as predicted.
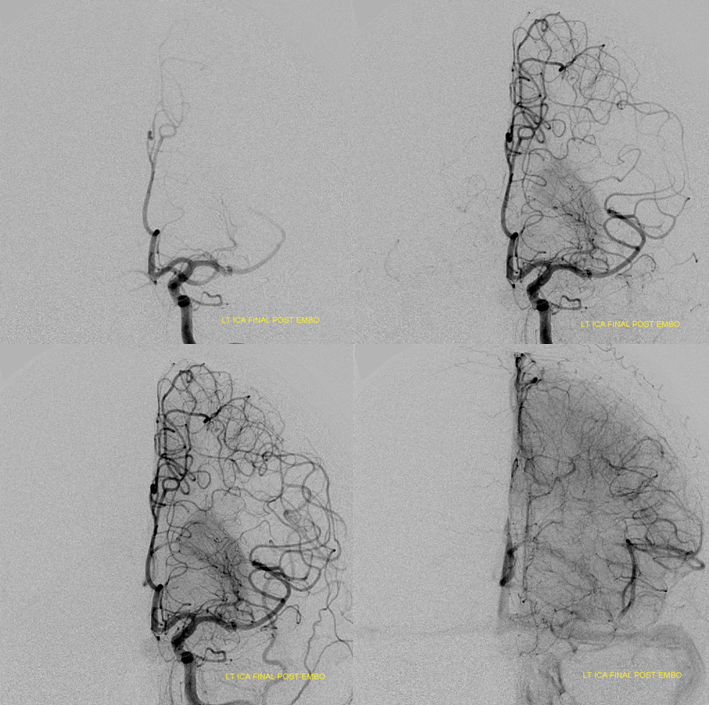
Additional segmental collaterals: pericalossal to posterior pericalossal, and PCA-MCA convexity
A relatively frequent venue of PCA-ACA collateralization, whereby the distal pericalossal artery connects with the posterior pericalossal branch of the PCA. This allows for reconstitution of either territory, sometimes to remarkable degree. In this patient with proximal left P2 occlusion, the PCA territory of the parieto-occipital convexity (dark green) is being reconstituted via ACA convexity vessels (bright green), and the P3 and more distal segments of the temporal/occipital region (red) via pericalossal (orange) connection to the posterior pericalossal (purple) system.
Robust posterior pericalossal-pericalossal collaterals: This connection is more fully developed here, helping reconstitute a substantial portion of the anterior cerebral territory.
The right ICA is occluded at the origin. The posterior pericalossal branches of the PCA (red) reconstitute the pericalossal territory of the ACA (yellow), with runoff into the anterior frontal branch (purple). The MCA/PCA parieto-occipital watershed has been substantially shifted anteriorly such that most of the parietal lobe is being perfused by the PCA (no arrows here, but similar to the case immediately below)
PCA-MCA convexity (leptomeningeal) collaterals: In this patient with supraclinoid left ICA occlusion, the left PCA supports both parietal convexity (red) and essentially the entire temporal lobe (green) via inferior temporal collaterals — a sizeable takeover of MCA territory (PCA typically supplies mesial and variable territories of inferior temporal lobe. Essentially, the PCA-MCA temporal watershed has been shifted to the operculum.
OTHER COLLATERALS DISTAL TO THE COW
Moya-Moya: Another example of collateral circulation, with a “rete” type arrangement supporting MCA territory in cases of M1 segment occlusion — a condition with multiple causes, and often seen in youth. An angiogram of the same patient as in the above MRI is shown below.
Another patient, same as above in case of PCA-MCA temporal reconsitution. Yellow arrows denote the “rete” moya moya system. Notice prominent Anterior choroidal perforator branches (pink) forming part of the reconsitution. The perforator branches from all vessels are typically involved(MCA, choroidal, A1, etc.
Another collateral route…
COLLATERALS AT THE CIRCLE OF WILLIS
The COW system is, in principle, the primary source of anatomical collateral support for the intracranial circulation. A well-developed ACOM can support an entire hemisphere when the ICA is occluded and no functional PCOM exists. The adequacy of support can be estimated via a balloon test occlusion (see above)
In this patient with neck squamous cell carcinoma and carotid blowout (green arrows show ulcerated carotid, radiation seeds are in purple), the left carotid artery has been closed with multiple coils. The ACOM (red) through the A1 (blue) fully supports the left hemisphere without hemodynamically significant delay in transit over the convexity.
ACOM reconstitution of left ICA territory, with Moya-Moya Component.
Patient with supraclinoid ICA occlusion — ACOM reconsitutes MCA/ACA territory. However, notice that the left M1 segment is deficient, in a Moya-Moya type pattern (yellow). Likely because of this, the normal MCA/ACA watershed is shifted inferiorly (red arrows) and there is signficiant delay in transit at this region. Patients such as this one have a very low tolerance for hypotension.
It is better to judge adequacy of perfusion, or “delay”, on venous phase rather than arterial phase images. This is because in the arterial phase the “filling out” of the territory at risk may be delayed while unopacified region may not become apparent until later in the imaging phase. Once the “control” hemisphere is in venous phase, the “test” half can be evaluated against a full timetable of contrast transit through all brain phases. Furhtermore, it allows for better time quantitation of delay as more images of similar phase are available on the conrol side. On the bottom left image, for example, the right hemisphere is in early venous phase, while the right inferior convexity is still arterial — a signficiant delay. On the bottom right image, the late venous phase of the right hemisphere is contrasted with the capillary phase of the left inferior convexity.
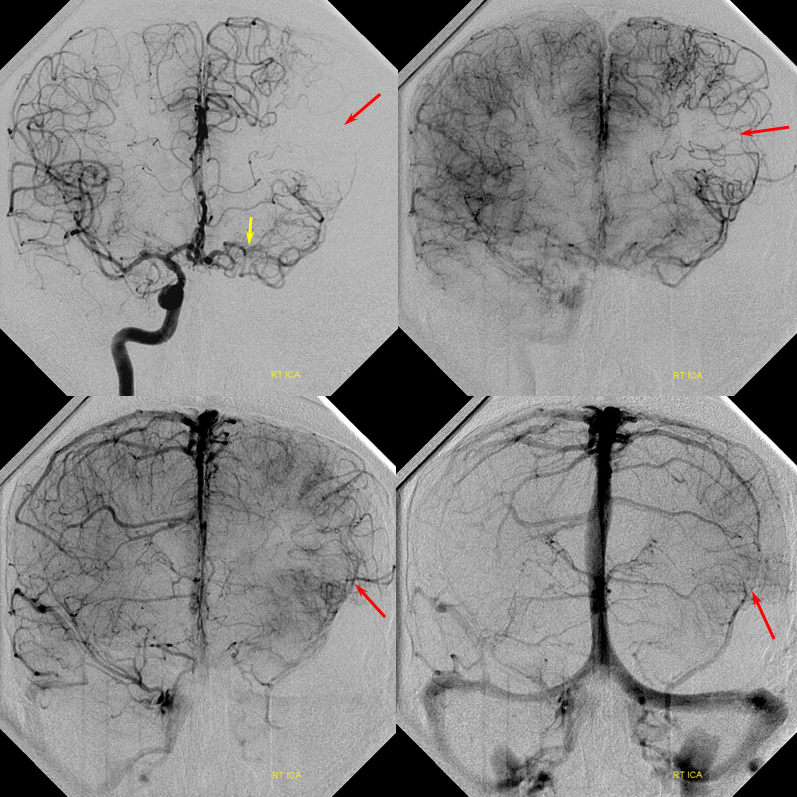
PCOM to anterior circulation — another common route of ICA territory reconstitution via basilar/PCA route through retrograde PCOM flow. PPCOM=red; MCA=yellow; cortical MCA branches = light blue; occluded left ICA=green; patent right ICA=pink
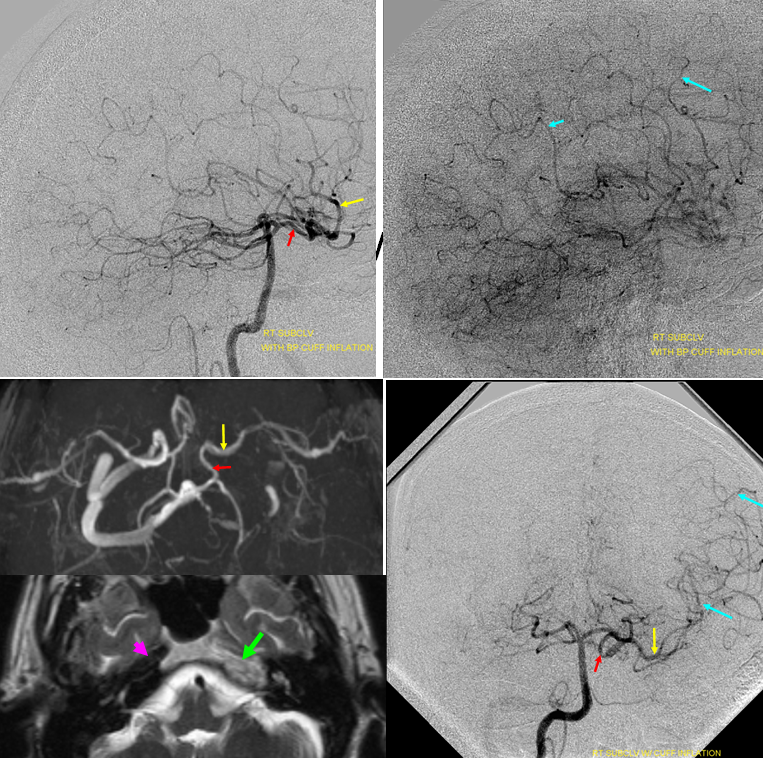
Extracranial to intracranial collateral circulation pathways.
Proximal to the COW, in setting of ICA occlusion, the ophthalmic artery, secondary to its myriad connections with the facial, internal maxillary, and superficial temporal systems, is most commonly recruited to reconstitute ICA flow in regrograde fashion. Other robust sources of ICA re-supply come from pathways related to the Inferolateral Trunk(ILT) and less commonly the Meningohypophyseal trunk(MHT) and mandibulovidian artery. These can be visualized on MRA when substantially well-developed.
Ophthalmic artery — for main discussion of this vessel, see our dedicated Ophthalmic page.
Imaging:
Figure 4: Pictorial visualization of the “classic” Ophthalmic Artery, intracranial view above and orbital view below.
A) Main Ophthalmic Artery
B) Lacrimal branch (actually many small branches by the time it gets to the lacrimal gland), which will collateralize with IMAX anterior deep temporal branches (K) thru transosseous (M) or muscular (L) routes. This is a very common pathway of ophthalmic / ICA reconstitution via the IMAX.
C) Medial division which gives off important anterior (G) and posterior (H) ethmoidal arteries. These enter the anterior cranial fossa thru respective foramina and supply regions of the cribriform plate and anterior falx. The anterior falcine artery can be particularly prominent especially after pterional craniotomies or other destructive processes of the middle meningeal artery. The ethmoid arteries also send branches into the nasal cavity (see below)
D) Central retinal artery: a true end-artery with NO collaterals. Occlusion results in irreversible permanent loss of vision. Aside from the central retinal artery, the other ophthalmic branches supply muscle and other orbital tissue.
E) Recurrent tentorial branch — an important collateral pathway connecting orbit to middle cranial fossa, collateralizing with anteromedial branch (I) of the ILT. Both (E) and (I) are vestiges of the primitive dorsal ophthalmic artery (see embryology)
F) Recurrent meningeal branch — important branch from the lateral (lacrimal) division which can collateralize with ophthalmic branch (J) of the middle meningeal artery and is a potential MMA to ophthalmic connection, often visualized in setting of main ophthalmic or ICA occlusions. This artery tends to exit the orbit through its own foramen, which when large enough carries a name of Foramen of Hyrtl
F1) Anterior Frontal Meningeal Branch — vascularises dura of the frontal convexity, can be prominent in setting of meningiomas, etc.
N) Inferior branches supplying muscle and other tissue, which can collateralize with distal inferior orbital branch (O) of the IMAX exiting through the infraorbital foramen and angular branch (P) of the facial artery.
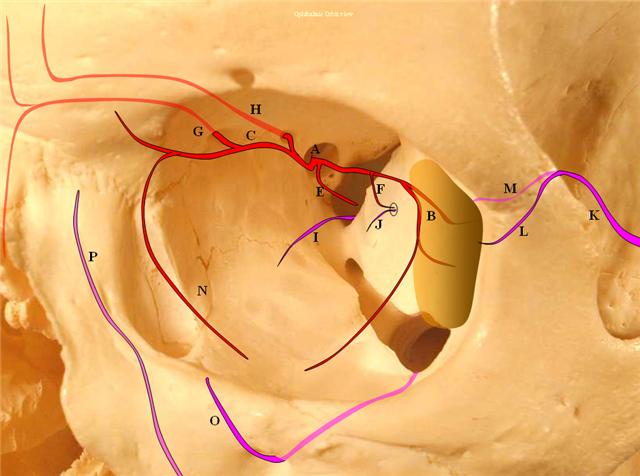
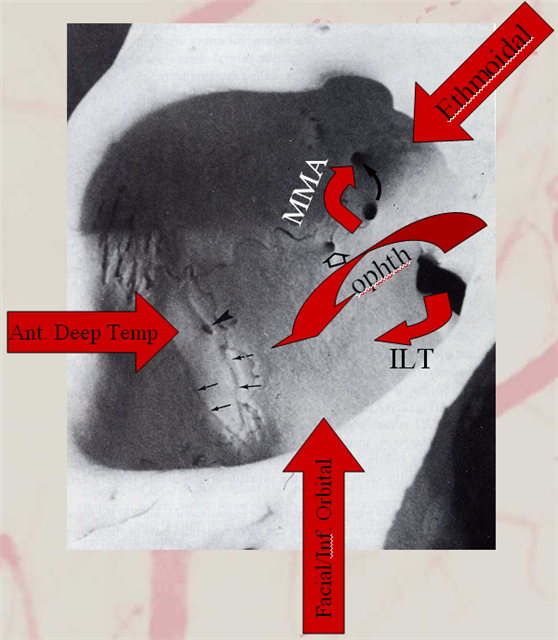
Collateral pathways into/from the orbit. Orbital photo from Lasjaunias, Berenstein, and Ter Brugge. Surgical Neuroangiography, 2nd edition, 1st volume, page 446. Bony landmarks attest to collateral potential of the orbital vasular supply. The meningolacrimal branch of the MMA enters the orbit through foramen of Hyrtl (open arrow). The MMA can enter the orbit (meningoophthalmic branch, curved arrow) The anteromedial branch of the ILT can collateralize through the superior orbital fissure. The anterior deep temporal branches can be transosseous (arrowhead) or muscular draped over the lateral orbital wall and get into the orbit that way to hook up with lacrimal branches of the ophthalmic. Angular branch of the facial artery or the infraorbital artery collateralize the orbit from below. Finally, the ethmoidal arteries, usually supplied by the ophthalmic to vascularize crista galli and anterior falx and superior nasal cavity can on occasion reverse flow and backfeed into the ophthalmic, although other collaterals are much more frequently recruited for this purpose.
Opthalmic artery reconstitution of the ICA
The opthalmic artery represents the most common pathway of retrograde reconstitution of the ICA following a more proximal acquired occlusion. All of the above depicted ECA to ophthalmic anastomoses can participate in suppying the ophthalmic artery, which reverses flow into the ICA and reconstitutes brain parenchyma. Statistically, the most frequently recruited collateral routes are through the anterior deep temporal artery and middle meningeal artery, with less important contributions from the angular and infraorbital channelsand. Other common pathways of ICA reconstituion not involving the ophthalmic artery utilize the ILT via the IMAX (usually foramen rotundum, MMA thru its cavernous and sphenoid ridge branches, and accessory meningeal thru foramen ovale) Below are some examples:
Ethmoidal and Anterior Deep Temporal Reconstitution of ICA
AP projection (above) and Lateral (below) demonstrating complete Right ICA occlusion. The ophthalmic artery retrogradely reconstitutes ICA flow through anterior deep temporal collateral to the lacrimal branches and sphenopalatine IMAX branches collateralizing with ethmoidal branches of the anterior and posterior septum (a.k.a. red and orange arrows of the case immediately above)
ICA occlusion with ILT and ophthalmic reconstitution. The sphenoidal branch (green, best seen on AP and foreshortened on the lateral) of the MMA (yellow) reconstitutes the ophthalmic artery (Blue). Cavernous branches of the MMA (red) also participate in reconstitution of the inferolateral trunk region (brown)
Lateral and AP views of another case showing robust retrograde flow in the ophthalmic artery (red) from sphenoid brach (yellow) of the MMA (blue) and from septal branches of the distal IMAX into the ethmoid branches of the ophthalmic (purple)
Noninvasive imaing of ophthalmic ICA reconstitution: Present day TOF MRA on a 1.5 T machine can be quite effective in visualizing these collaterals especially when one knows where to look for them. In this patient, multiple ECA-ICA collaterals can be seen on MRA and same vessels demonstrated on angio.
Three MIP and one source MRA images (top) and AP and lateral angiogram views of the same patient with left ICA occluson and vasa vasorum reconstitution. The same collateral pathways can be seen on both studies. Pink=occipital artery. Brown = vert. Green = MMA; light blue = sphenoid branch of MMA; yellow= foramen rotundum branch of IMAX; Red = cavernous branch of MMA
CASE 2
Standard projection maxiumim intensity (MIP) projection MRA images demonstrate bilateral intracranial ICA occlusions. The left vert and basilar are tortuous and prominent. Both PCOMs are large and undoubtedly help reconstitute ICA territory. Notice also the tortuosity and prominence of vessels in bilateral ophthalmic regions (red arrows), attesting to presence of robust ophthalmic ECA-ICA anastomoses. The largest of these is a markedly hypertrophied right MMA (yellow arrows)
Targeted MIP images highlight the course of the right MMA (red arrows), which reconstitutes the right carotid at the ophthalmic segment through the artery of the sphenoid ridge (yellow arrows). Other IMAX non-ophthalmic collaterals are also present.
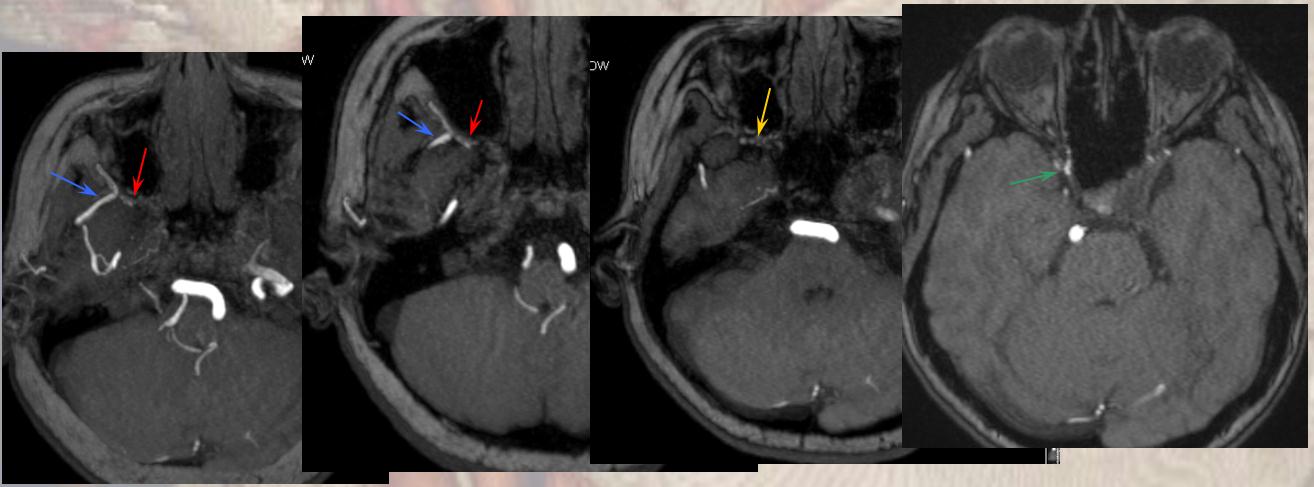
Another likely route is from the distal internal maxillary within the prerygopalatine fossa. This IMAX (blue arrow) takes a superficial course relative to the lateral pterygoid muscle, and gives off a medially-oriented branch (red arrow) which likely connects to a faintly seen area of flow-related signal in the region of the pterygopalatine fossa (yellow arrow), and extending posteriorly towards the lateral border of the cavernous sinus (green arrow). This is most probably a foramen rotundum branch-ILT anastomosis (see ILTas well)
Inferolateral Trunk — for main discussion of this vessel, see our dedicated ILT page.
The ILT arises as a single trunk or a collection of vessels from the lataral aspect of the cavernous carotid artery region. It usually supplies adjacent dura and cranial nerves and has extensive anastomoses with the extracranial circulation, particulary branches of the IMAX and middle meningeal artery as well as ophthalmic artery. It is a remnant of the primitive dorsal ophthalmic artery. The ILT and adult ophthalmic arteries are primary routes of ICA reconstitution following proximal ICA occlusion. The importance of understanding ECA-ICA collaterals of the ILT is paramount. Common routes of ILT inflow include foramen rotundum branch, accessory and cavernous branches of the accessory and middle meningeal arteries.
Figure 1: ILT and important anastomoses:
A) ILT
B) Recurrent branch of the ILT — courses along CN IV, and colalteralizes with branches of the MHT. Not rarely it annexes the territory of marginal tentorial (G) or lateral tentorial (F) arteries which more commonly come off the ILT (see ILT).
C) Anteromedial branch — a very important branch, this is a vestige of the primitive dorsal ophthalmic artery which in very early embryonic life supplied the orbit together with primitive ventral ophthalmic artery. This artery is hemodynamic balance with the recurrent meningeal branch (N) of the ophthalmic artery (M). Very rarely, when proximal ophthalmic (M) is absent, this branch (C) can reconstitute the ophthalmic artery (see ophthalmic artery section), although more commonly the middle meningeal artery fulfills this role through its opthalmic branch (K)
D) Artery to the foramen rotundum — another very important vessel supplying the nerve of the same foramen. It collateralizes with foramen rotundum branch of the IMAX (L), and is the primary route of ICA reconstitution via the IMAX
E) Foramen ovale branch — yet another important branch which supplies the appropriate foramen ovale nerve and collateralizes with the accessory meningeal artery (J) and also with carotid branch of the ascending pharyngeal artery (H) coming up through foramen ovale and cavernous branch (I) of the middle meningeal artery.
The checkered vessel anastomosing with the basilar artery (N) is the trigeminal artery, which sometimes originates from the region of the ILT.
Example 1
ICA reconstitution following iatrogenic occlusion with coils (orange arrows) The ILT reconsitutes the ICA via sphenoid branches of the MMA (purple), foramen rotundum branch of the IMAX (yellow) and cavernous branches of the Accessory Meningeal (blue) arteries. The patient originally presented with a dural CC fistula. Possibly because the ILT vessels were already enlarged, they were relatively quickly recruited into ICA supply instead of the ophthalmic artery (green) which continues to fill its usual anterograde direction, highly unusual for an ICA occlusion. The coils in the cavernous sinus are also faintly visible in the region between the red and yellow arrows on the lateral projection (see below as well)
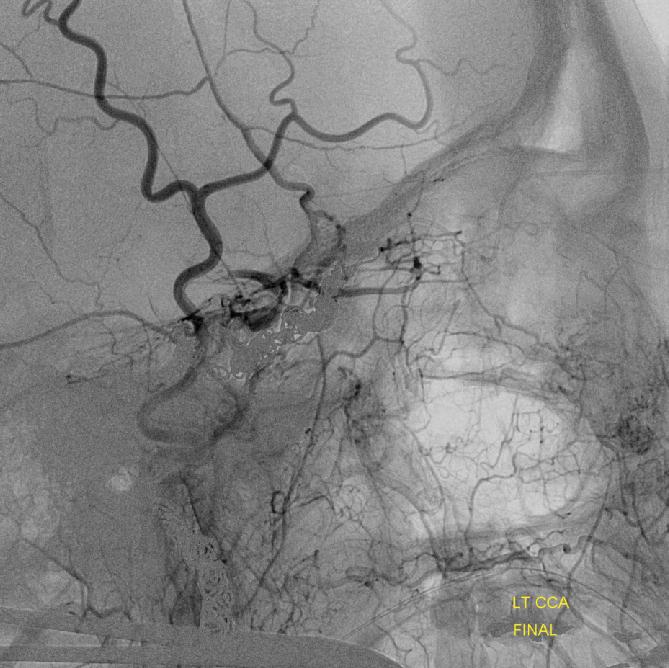
Example 2
ICA occlusion with ILT and ophthalmic reconstitution. Cavernous branches of the MMA (red) participate in reconstitution of the inferolateral trunk region (brown) The sphenoidal branch (green, best seen on AP and foreshortened on the lateral) of the MMA (yellow) reconstitutes the ophthalmic artery (Blue)
Accessory meningeal reconstitution of the MCA territory via foramen ovale branch — right ICA is occluded at the origin. The ACOM is insufficient. Brisk reconstitution of the MCA territory is afforded via a the accessory meningeal artery inflow via the foramen ovale branches. Additional supply to the cavernous carotid was present via posterior clival branches of the MHT (see MHT). The orbital region is being reconstituted via the MMA.
Red=carotid; purple=extracranial MMA, pink=foramen spinosum; yellow=intracranial MMA; light blue=sphenoidal branch; dark blue=meningo-ophthalmic artery; green=accessory meningeal artery; white=foramen ovale branch of the ILT; brown=carotid stump
Temporal and MMA ICA reconstitution pathways
A relatively unusual collateral sourse from the supratrochlar branches (yellow) of the superficial temporal (red) artery to reconstitute the ophthalmic (pink). The carvernous branch (purple) of the MMA artery (white) reconstitutes the cavernous ICA at the level of the ILT (light blue)
light blue).
Same patient after an STA-MCA bypass. The STA (red) has been disconnected from the ophthalmic and anastomosed end-to-side (yellow) to anterior parietal branch of the MCA. Yes, that’s an iatrogenic collateral.
Infrequently seen even with modern angiographic equipment, the MV artery originates from the petrous portion of the Internal Carotid artery. The artery, typically, consists of two branches — the Mandibular and the Vidian. Either or both branches may be present separately.
Both Mandibular and Vidian branches originate from the ascending petrous portion of the ICA in the foramen lacerum. The Vidian branch courses forward to enter the Vidian canal (together with the greater superficial petrosal nerve) and exits into the pterygopalatine fossa, where it meets the Internal Maxillary Artery. Think of the Vidian is a thin connection between the much larger ICA and IMAX.
VIDIAN BRANCH
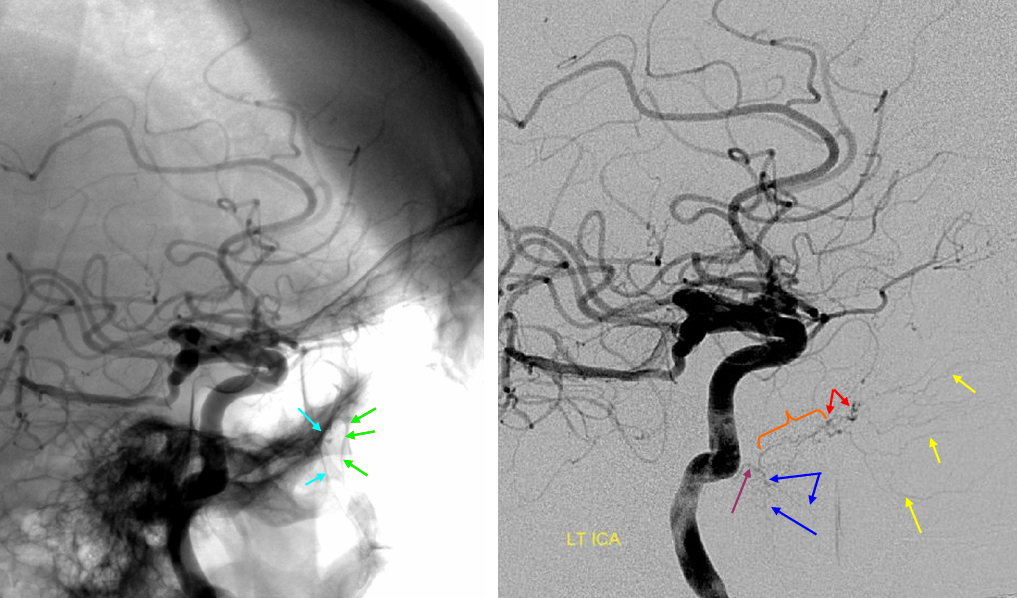
Native and subtraction Lateral (top) and AP (bottom) views of a particularly prominent mandibulovidian artery in absence of pathology. The proximal trunk is labeled in purple. The Vidian artery portion inside the canal is orange and projects above the mandibullar portion. The Vidian canal opens into the posterior portion of the pterygopalatine fossa which is a consistent landmark (blue arrows back wall, green arrows front wall). The part in the fossa is marked with red arrows. In this case, the artery supplies extensively the nasal septum (yellow arrows). Typically, the nasal septum is supplied by sphenopalatine artery branches of the IMAX, which in this case is hypoplastic. The mandibullar branch is labeled with blue arrows and supplies tissues adjacent to the mandibullar condyle.
Vidian IMAX Branch — the more typical origin of the Vidian artery, it projects posterior to the termanal IMAX portion in the pterygopalatine fossa.
The Vidian artery (red) is a small branch projecting posterior to the terminal IMAX. The foramen rotundum branch also projects posterior but would be pointing more rostral towards the cavernous portion of the carotid artery. Notice prominent ethmoidal branches of the ophthlamic artery (purple) and the more typical supply of the nasal septum and turbinates via sphenopalatine branches of the IMAX (yellow)
yellow)
Sequential axial CT images through the Vidian canal (red) connecting the proximal petrous carotid ICA (purple) to the pterygopalatine fossa (yellow). The pterygopalatine fossa on the left is enlarged by a Juvenile Angiofibroma. The vidian artery did not however participate in supply of the lesion, as seen on a pre-operative embolization angiogram (right).
ICA reconstitution via the Vidian Branch
This is a relatively infequent route of ICA reconstituion which can be important in setting of proximal disease or, in this case, balloon test occlusion.
From Jason W. Allen, M.D., PH.D., Anthony J. G. Alastra, M.D., and Peter K. Nelson, M.D. Proximal intracranial internal carotid artery branches: prevalence and importance for balloon occlusion test. Journal of Neurosugery 2005; 102(1) 45-52.
“Sequential DS angiograms images obtained during the initial BOT in the midcervical left ICA following injection of contrast material through the central lumen of the balloon catheter. Despite the arrest of antegrade flow within the cervical ICA immediately beyond the inflated balloon (black arrows), prompt washout of the contrast agent from the more distal petrous (white arrowhead) and supraclinoid (white arrow) segments of the ICA is evident based on the collateral inflow of unopacified blood through anastomoses with the vidian and ophthalmic arteries, respectively.” Figure and description courtesy of Dr. Peter Kim Nelson.
Ascending pharyngeal Artery reconstituion of both vertebral artery and internal carotid artery in a patient with M1 dissection via the Vidian branch. A child with spontaneous right M1 dissection. Right ICA runoff is diminished and all dural branches are well-seen. The mandibulovidian artery (red) is prominent and is briskly opacified via the AP injection through the superior pharyngeal division (bottom set of pictures). There is also collateral connection with the vertebral artery present via the odontoid arch.

Red=mandibulovidian branch; Orange=mucosal blush of the posterior nasopharynx; dark blue=Ascending Pharyngeal, superior division (contralateral side also visible on the AP ascending pharygeal projection on bottom left); Purple=ILT; Pink=MHT; Black=pituitary blush; Yellow=PCOM; White=anterior choroidal; Green=anterior meningeal (off ophthalmic, origin outside field of view on the lateral ICA projection); Double Green=odontoid arch of the neuromeningeal division of the ascending pharyngeal artery; Double Red=vertebral artery.
Contralateral Ascending pharyngeal to vidian artery — can’t get too excited about this one. The right ICA is occluded above the bifurcation. Petrous ICA is re-constituted via bilateral ascending pharyngeal arteries (superior pharyngeal division) converging onto the mandibulovidian artery.
The RIGHT ICA is occluded at the neck. Red=ascending pharyngeal. Yellow=vidian. Pink=petrous ICA segment. Green=ophthalmic. Light blue=ophthalmic artery ICA segment. White=ethmoidal branches; purple = sphenoidal branch of the MMA. Dark green=contralateral LEFT ascending pharyngeal superior pharyngeal division.
Extracranial to intracranial collateral circulation — Sugrical Synangiosis.
A unique pathway for extracranial to intracranial collateralization often develops in patients with slowly progressive occlusive disease. An autosynangiosis refers to parasitization of extracranial supply by the brain. Typically, this inloves recruitment of dural arterial vasculature by the subjacent pial surface of the brain. The mechanism is more often observed in younger patients, such as those with Moya-Moya disease. A surgical synangiosis exploits this mechanism by direct application of various forms of temporalis muscle flap or dural onto the brain to promote the same process. An even more basic approach is to drill burr holes through the skull, which induces hyperemia and often results in formation of synangiosis.
Arterial and capillary phase angiographic injection of the left ICA, demonstrating moya moya changes, with relative ICA hypoperfusion of the posterior frontal and parietal convexities.
External carotid injection of the same patient, demonstrating osseous changes of synangiosis with craniectomy and burr holes (green arrows). Multiple MMA branches (orange) reconstitute convexity branches of the middle cerebral artery (red), resulting in extensive support to the posterior frontal, parietal, and occipial lobes.
LT Common Carotid Injection, showing overall balanced delayed filling of the cortex by combination of ECA and ICA
Autosynangiosis
Surgical Synangiosis is essentially an augmentation of “naturally” or spontaneously occuring synangiosis, developing in responce to a relatively long-standing vascular constraint. This process is more robust in younger patients, and so is often encountered in angiographic evaluation of Moya Moya disease. In this 4 year old patient, an impressive autosynangiosis recruiting the middle meningeal artery developed to supply mesial posterior frontal and anterior parietal lobes, in response to progressive narrowing of right ICA and left A1 segments.
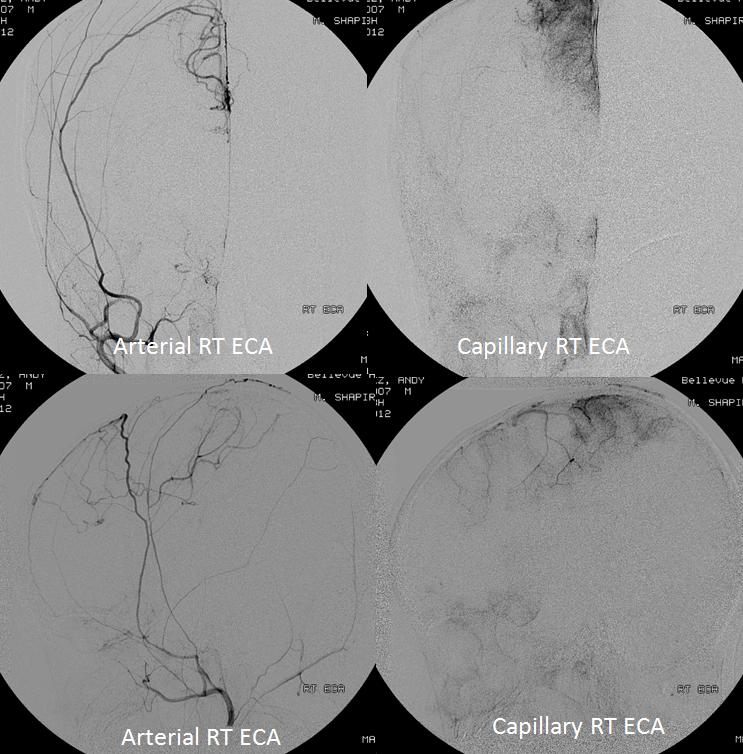
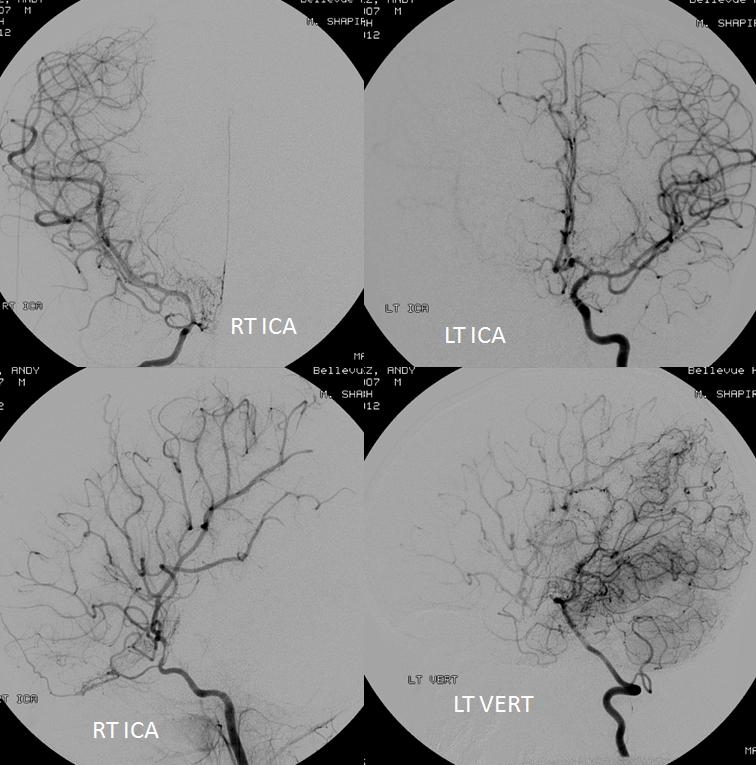
One of the key factors in selection of collateral pathways is the site of primary occlusion. Collaterals can only develop downstream from the occlusion site. For example, occlusion of the cervical ICA often results in ophthalmic, ILT, and MHT participation, not to mention the PCOM and ACOM. However, if the carotid is closed at the level of the terminus (as in cases of moya-moya), none of these routes are going to be effective since they are all located proximal to occlusion site. For each occlusion level, a set of “usual suspects” collateral pathways exists, — ways that are preferentially recruited to reconstitute the territory at risk. In case of carotid terminus occlusions, these pathways are leptomeningeal for PCA/MCA and PCA/ACA territory, and dural – pial in cases of auto-synangiosis, and to some extent posterior pericalossal-anterior pericalossal routes. There is nothing specific about the particular collateral pathway in terms of making a diagnosis of the specific cause of occlusion — but pattern of collateralization does strongly reflect the location of primary vessel failure, and for each location there are the usual suspects (atherosclerotic disease for proximal ICA, dissection for distal ICA, intracranial atherosclerosis for intracranial ICA especially at cavernous and supraclinoid segments, moya-moya at carotid terminus, and various external mass effect lesions depending on their location (sphenoid wing meningioma and supraclinoid carotid occlusion, for example)
In the following patient, a giant shenoid wing meningioma resulted in occlusion of both supraclinoid ICAs, similar to a moya-moya pattern. Therefore, the primary method of reconstitution is via leptomeningeal PCA-MCA (purple arrows) and PCA-ACA (light blue arrows) collaterals, the posterior to anterior pericalossal (yellow) anastomosis, and left more than right middle meningeal artery auto-synangioses with the MCA territory on the left (motor strip, purple oval) and right MMA to left ACA territory as well (white arrows). Notice meningioma tumor blush (orange oval)
Below are the cross-sectional images from the same patient:
Anterior spinal artery collateral route
Sometimes, this is the way to go. Anterior spinal can very prominently participate in resupply of intracranial vertebral and basilar arteries, as in this case, where vert occlusion distal origin of PICA is associated with robust and fully adequate reconstitution of basilar artery via the anterior spinal artery.
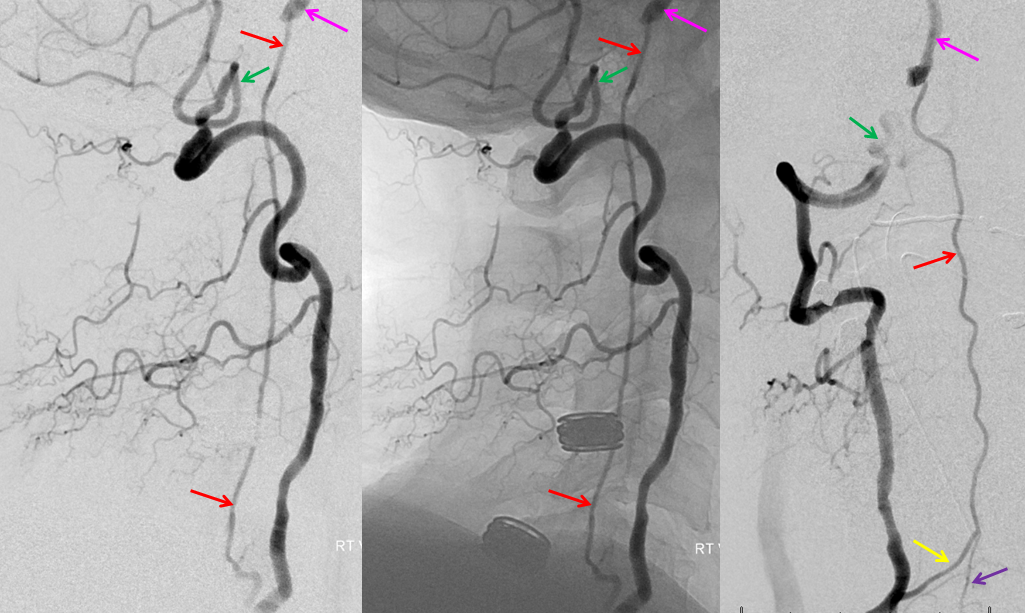
Lateral cranial view, lest you think the ASA is inadequate
Extracranial to extracranial collateral pathways.
ICA to ICA reconstitution via vasa vasorum
A somewhat under-recognized, curious instance of ICA reconstituting itself has been occasionally observed. A completely closed ICA has occasinally been seen to “re-open”, whereas in fact the native lumen of the ICA remains closed, while small arterial channels within the carotid wall (vasa vasorum) gradually hypertrophy to effectively bridge the closed arterial segment. The end result is a somewhat tortuous and irregular appearance of what looks like cervical ICA, but in fact is a new channel within what was previously the carotid wall. To those partial towards differential dagnoses, the look may suggest possible fibromuscular dysplasia; on closer inspection it is clear that the apperance is quite unlike FMD, however. We have seen cases of this kind of vasa vasorum disposition, both in setting of reported ICA re-canalization and in evaluation of pathologic arterial conditions. Below are several examples:
Patient 1, below, was referred for evaluation of bifrontal deep white matter infarctions in setting of sickle cell anemia. Tortuous corscrew appearance of the cervical “carotid” (red arrows) which in fact represents a dilated vasa vasorum channel, reconstituting normal caliber and morphology cavernous segment (yellow arrows)
For nonbelieves, search vasa vasorum in Medline.
Common carotid occlusion
In such instances, collateral circulation often comes from the external carotid artery to reconstitute the carotid bifurcation and subsequently the ICA. The occipital is most commonly recruited to re-establish external carotid artery flow because of its extensive segmental connections to the three longitudinal cervical systems — the deep cervical, vertebral, and anterior cervical arteries. Typical routes include vertebral to occipital, deep cervical to occipital, or anterior cervical to occipital routes. In the example below, the left common carotid artery and proximal left vertebral artery are occluded. Injection of the left subclavian artery demonstrates a large deep cervical artery (purple) which, through C2, C3, and C4 segmental collaterals (brown) reconstitutes the distal cervical vertebral artery (yellow) thus supporting the PICA component of the posterior circulation (not shown). Additionally, the deep cervical, through a huge connection to the occiptal artery (orange), reconstitutes the carotid bifurcation (light blue) and subsequently the markedly irregular internal carotid artery (red), maintaining antegrade ophthalmic artery flow and even opacifying briefly the left M1 segment (rightmost angiogram, above the top red arrow).
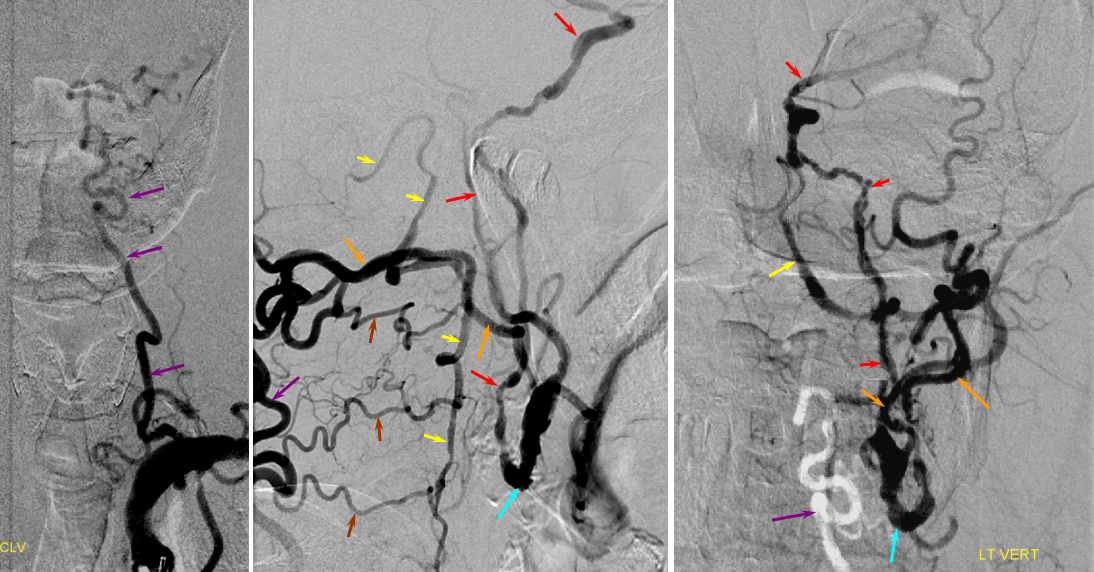
Inferior Thyroid to Superior Thyroid Collateral Reconstitution of the ICA
There is no limit to the collateral potential of the body, especially given time. This case is more than a curious rarity — it illustrates the potential for tissue level reconstitution. Thyroid and other highly vascular organs have richly interconnected capillary and precapillary networks and can very efficiently resupply themselves in cases of all but extreme constraints. Who ever heard of thyroid gland necrosis due to ischemia?
In this patient, a humongous clot (black arrows) extends across the entire left CCA: A reduced caliber ICA (red) is reconstituted by streams of contrast at the distal edge of the clot (orange). The proximal CCA stump is in yellow.
The patient is doing fine, thanks to a large ACOM. It is always best to estimate angiographic collateral reconstitution in the venous phase of the control side (image on right). Also notice nice MHT-to-MHT anastomoses (white) across the clival arcade (green), with ascending clival branches (purple) of the Ascending Pharyngeal Artery.
The internal carotid artery (red) is primarily reconstituted through the ECA (purple) at the level of the carotid bifurcation, in this case via the vertebral artery C1 muscular branch (white) anastomosis with the Occipital artery (brown). This recreates the Proatlantal type 1 disposition — Proatlantal artery is nothing but Occipital to vertebral anastomosis at C1 level. Other examples are found on the Occipital Artery page and on this page (vide supra) also.
Later images, in thyroid parenchymal phase, show superior thyroid (yellow) participation in ipsilateral ICA (red) reconstitution at the level of its proximal external takeoff.
Extracervical collaterals
In some particularly severe instances involving great vessel occlusion or disease, very distant collaterals are brought into play to reconstitute cervical and cerebral vasculature. These can be seen, for example, in setting of Takayasu’s arteritis which can lead to occlusion of all great vessels. These can be quite challenging to put together anatomically. Very importantly, noninvasive imaging can be extremely helpful in these cases because of the myriad collaterals involved. Angiograhically, investigation of each one provides a piece of the puzzle, whereas a global view of, for example, a contrast arch MRA, can both clarify the overall picture and direct further catheter investigation, should the latter be necessary.
The collaterals typically recruited for reconstituion of arch occlusion are 1) thoracic segmental vessels and 2) internal mammary arteries. The thoracic segmental arteries, via the intercostal arteries, can effectively reconstitute the subclavian through retrograde flow in the long thoracic artery. This will provide for inflow into the vertebral artery if the ostium of the latter remains patent. Even if the vertebral artery is not accessible (because of extensive proximal subclavian disease), the subclavian artery can still reconstitute both vertebral and carotid arteries through the deep and anterior cervical collateral channels, which were already illustrated above. Supreme intercostal arteries can also be considered as segmental arteries in this sense. Finally, internal mammary arteries can be retrogradely supplied through the hypogastric axis, arising as far caudal as the external iliac artery (!), to help reconstitute the subclavian and external carotid systems.
The following case of a young woman with Takayasu’s arteritis illustrates some of the above points.
Stereo contrast MRA arch, demonstrating occlusion of all great vessel ostia. Contrast is present in the cervical vasculature, though the origin of the collaterals is not well seen on this image.
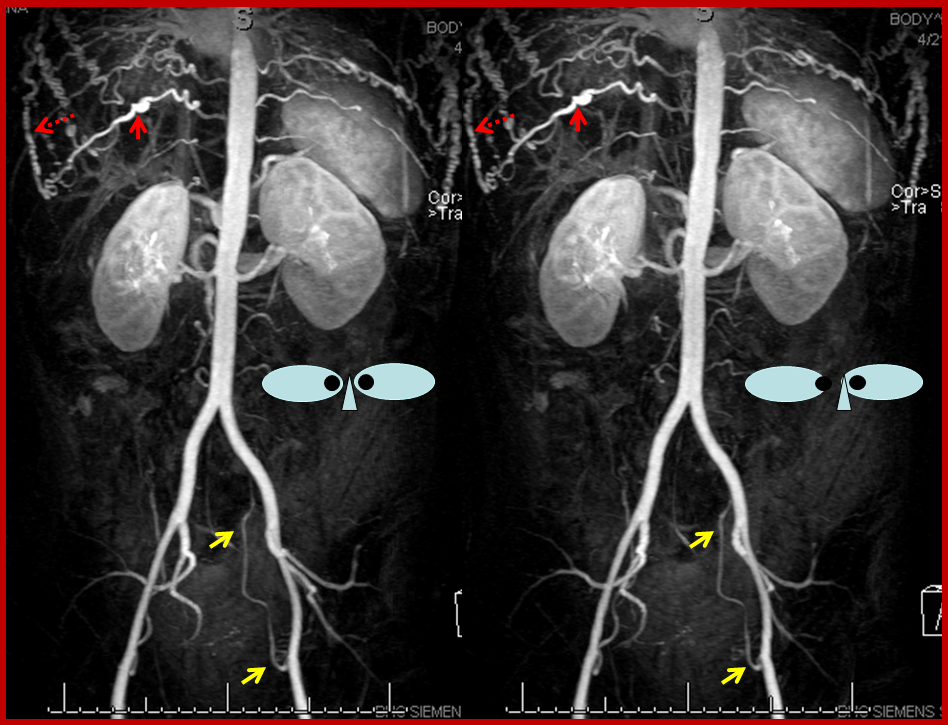
Stereo contrast MRA of the descending aorta. Markedly enlarged thoracic segmental/intercostal arteries (solid red arrow on one of them), reconstituting the long thoracic chain (dashed red arrow). Notice also enlraged inferior hypogastric arteries (yellow arrows), which will ultimately reconstitute the internal mammary arteries. This illustrates the importance of studying a global image such as this MRA, to plan out a catheter angiogram.
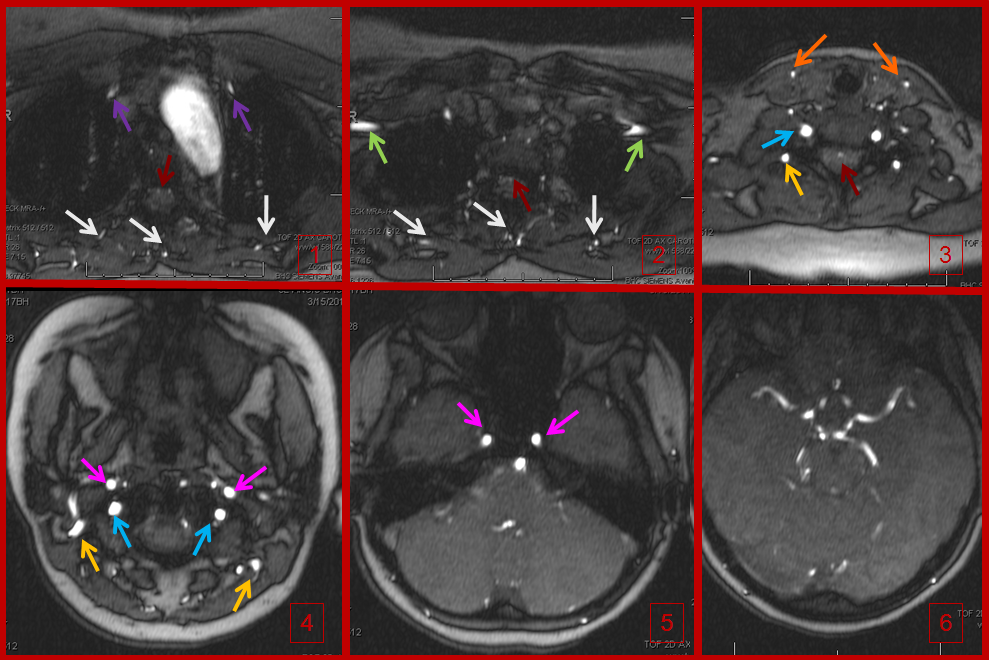
Time of flight (TOF) MRA of the neck and brain, demonstrating an intact circle of Willis (image 6). Images 1 and 2 demonstrate no evidence of flow in the great vessels. The internal mammary arteries (purple) are visualized, implying retrograde flow. The subclavian arteries (green) are visualized as well, via collaterals. Enlarged anterior spinal artery is present (brown arrow), undoubtedly helping reconstitute the vertebrobasilar system. Anterior cervical / thyroid arteries (orange arrows) and deep cervical arteries (dark yellow arrows) are also prominent. The internal carotid (pink) and vertebral (blue) arteries are relatively well reconstituted in the upper cervical segment. The common carotid artery is absent.
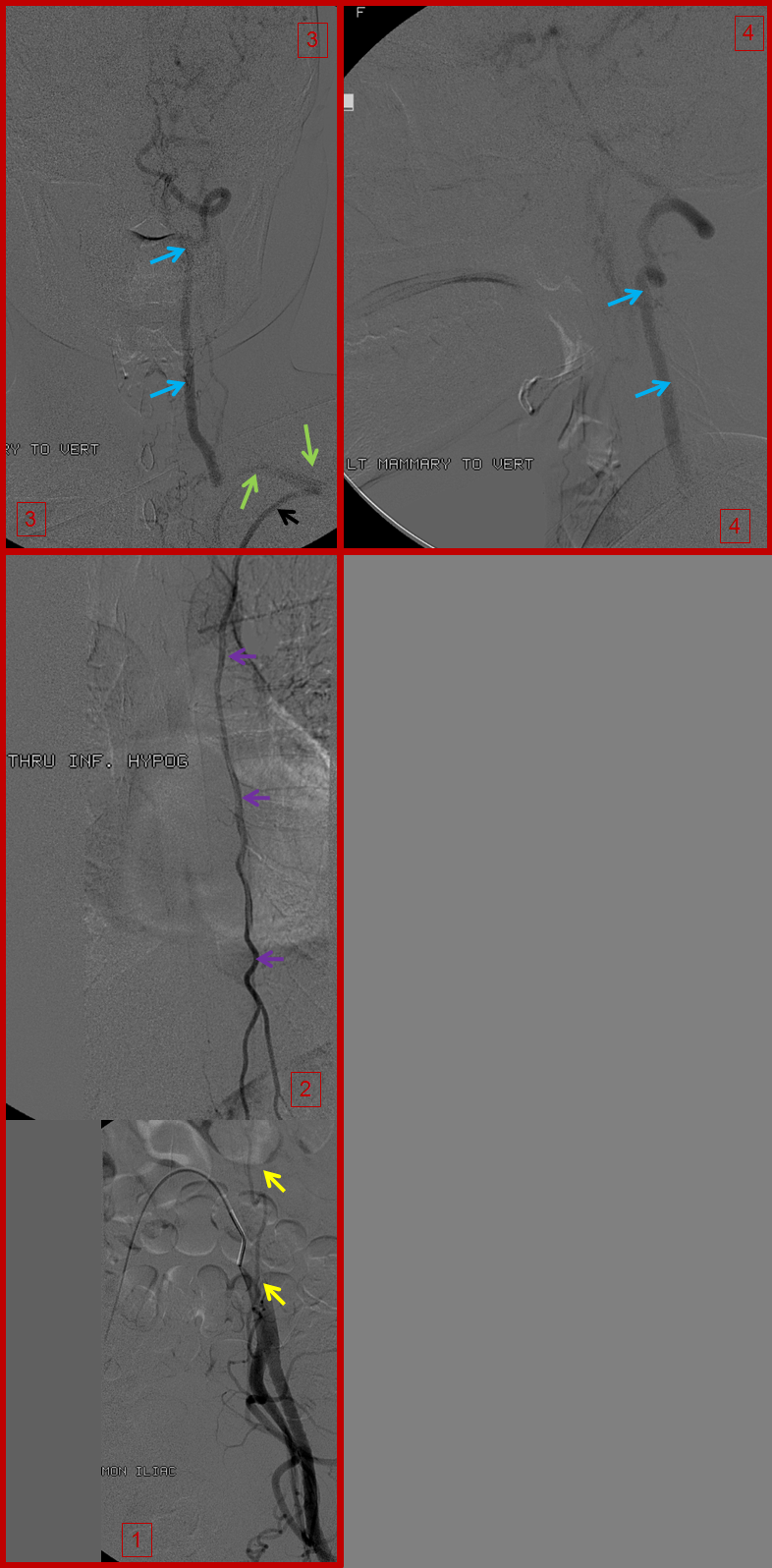
A composite picture of left inferior epigastric artery (yellow) injection (image 1), showing retrograde flow into the internal mammary artery (purple) over the chest (image 2), to reconstitute the subclavian artery (green) and ultimately the vertebral artery (blue), images 3 and 4. Notice, on image 4, retrograde flow in the posterior communicating artery (unlabeled), supporting the left anterior circulation.
Right inferior hypogastric (yellow) runoff, demonstrating the internal mammary artery (purple) over the chest on image 2, which reconstitues the anterior cervical / inferior / superior thyroid arteries (orange, image 3) and in this way ultimately supplies the internal carotid arteries (pink, image 4).
Composite image of left T9 segmental artery injection, reconstituting via an enlarged intercostal artery (solid red) the long thoracic artery (dashed red arrows), which retrogradely supports the subclavian (green) and vertebral arteries (blue). Notice stenosis of the subclavian artery just distal (anatomically) to the ostium of the vertebral artery.
A native image of left T8 injection.
Similar to left T8: Composite image of right T9 segmental artery (red) injection reconstituting, through the intercostal artery, the long thoracic artery (dashed red arrows) to support the subclavian artery (green) and vertebral artery (blue). A stenosis of the subclavian artery just distal (anatomically) to the vertebral ostium is also present
Finally, composite image of right T8 injection, demonstrating reconstitution of the deep cervical artery (orange) via collaterals along the posterior chest wall. Deep cervical artery effectively reconstitutes the vertebral artery (via C1-2) anastomoses and the internal carotid artery (pink) via the occipital artery.
Applications of collateral analysis — watershed infarction. Knowledge of the watershed concept allows one to take a reasonable guess at the location of lesion responsible for producing the watershed pattern. When an infarction in the watershed territory of two vascular beds is present, the responsible hemodynamic constraint is likely to be a single lesion, located proximal to the vessels making up the watershed territory AND distal to a collateral vessel which partially but not fully compensated for the ischemia. For example, an ACA-MCA territory watershed infarct implies that the lesion is located somewhere in the ipsilateral carotid system, and that some kind of collateral (ACOM, PCOM, ophthalimc, whatever) was recruited to supply perfusion to the core of ACA and MCA territories, but not sufficiently robust to extend its rescue to the watershed region. Simplistically, an MCA-ACA or MCA-PCA watershed infarction usually implies internal carotid artery stenosis. This is the information the clinician really wants to know — where to look for the lesion. The corrollary of watershed concept is the core/penumbra situation. An MCA occlusion, for example, produces a core of infarct in the deep MCA territory while the watershed region may in this case be spared, depending on extent of collateral supply from the adjacent vascular bed. The “watershed” region will therefore shift into the vacular bed previously subserved by the occluded segment, and a core will be established wherever the extent of collateral support reaches its limit.
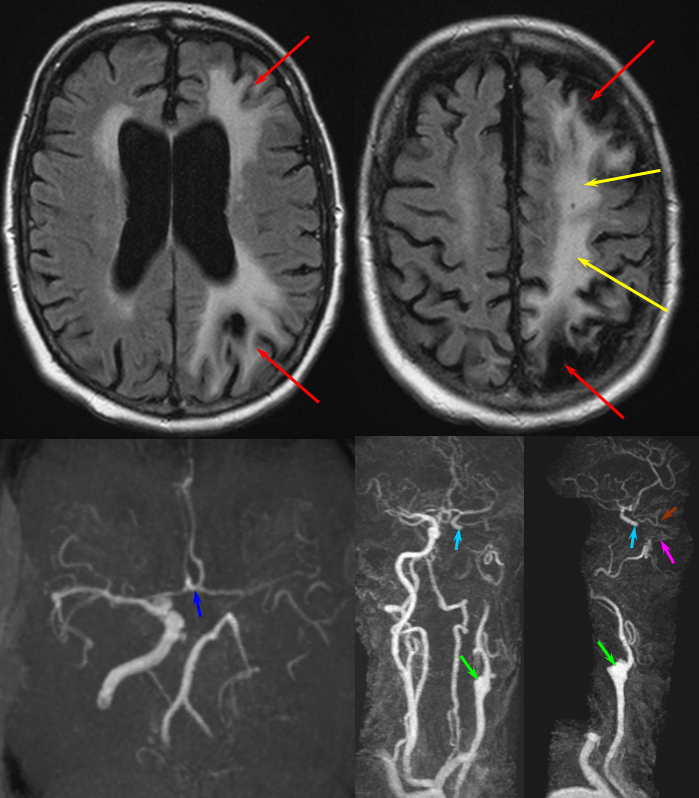
MCA-ACA and MCA-PCA territory watershed infarction (red) as well as deep MCA territory infarction (yellow) in a patient with occluded left ICA (green). The ACOM (dark blue) provided enough collateral support to maintain ACA and MCA territory to the exent possible; the watershed region markes its limit of hemodynamic re-supply. Notice suggestion of IMAX (pink) supply to the ophthalmic artery (brown) to revascularize the supraclinoid portion of the ICA (light blue)
ACA-MCA watershed infarct (red) in a patient status post carotid artery sacrifice with coils (purple) proximal to the ACOM (yellow)
Autoregulation and reperfusion
Compensatory vasodilatation is a common local response to inadequate perfusion. The vascular bed at risk undergoes variable degrees of vasodilatation depending on hemodynaimc need and individual disposition. This adaptive response comes at a price of potential hemorrage — the vasodilated territory looses its capacity for autoregulation and is not able to adequately adjust for variability in blood pressure. Hypertension, sudden or otherwise, may lead to hemorrhage in the risk territory. This is certainly true of dead brain (luxury perfusion of recently infarcted tissue and reperfusion hemorrhage) and is also true for non-infarcted tissue that is living on marginal support. The case below illustrates some of these concepts.
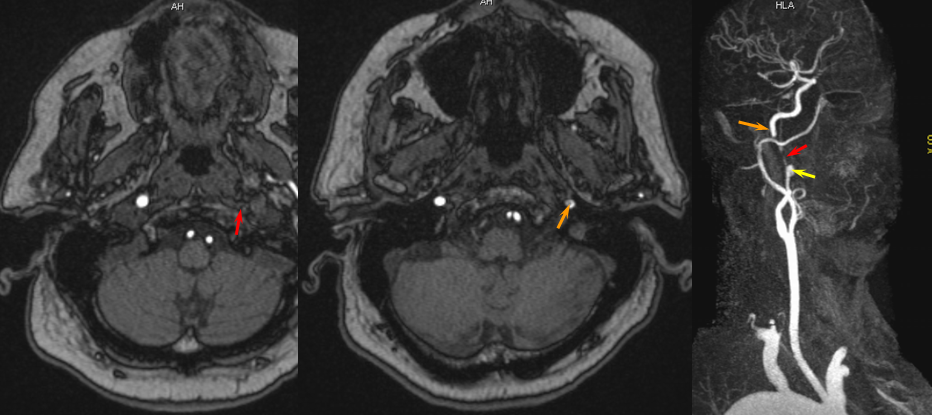
The patient presented with transint left eye obscurations and confusion/aphasia when standing, relieved by recumbency. This remarkable history illustrates the tenuous nature of the patient’s circulation. MRI/MRA demonstrates weak signal of left ICA at skull base (orange) on TOF MRA and stronger signal on contrast MRA. Visualization of ICA patency below the petrous segment should suggest a “string sign”, i.e. some patency of the vessel since there are usually no effective collateral inflow sourses at this level. A bulbous dilatation suggestive of a pseudoaneurysm is also present (yellow)
Angiogram of the same patient shows a “string sign” (red) in association with a pseudoaneurysm (purple). The convexity MCA territory (light blue) shows profound compensatory vasodilatation, in part because of inadequate collateral support from the contralateral ICA (dark blue) and vertebral artery (green) sourses.
Post-ICA stenting (tandem Precise carotid stents) shows tremendous vasodilatation (light lbue) of the mca convexity territory heretofore functioning on marginal pefusion, with a strong capillary blush (brown) almost similar to one observed in “luxury reperfusion” of dead brain. The patient’s pressures were maintained at 120 systolic or less immediately following stent placement to minimize the possibility of hemorrhage.
Illustrative cases:
Patient 1: In this patient with supraclinoid ICA occlusion, multiple pathways have developed that effectively serve as illustration of all kinds of collateral anastomoses. Among other routes, there is robust takeover of hte anterior and superior temporal love via normally much more modest temporal PCA branches. There is shift in the watershed with posterior temporal/parietal convexity vessels reconsituting normally MCA-supplied parietal territory. A relatively large ACOM supports the majority of ICA territory, with notable delay in the region of the MCA-ACA watershed over the frontal convexity — potentially a region of stoke in setting of hypotension for this patient. Also notice occlusion of the left M1 segment, with a Moya-Moya pattern. Finally, a middle meningeal synangiosis (parasitization of dural/pial extracranial supply by the brain in setting of chronic ischemia) minimally helps support portion of the parietal cortex.
The ICA is occluded at the supraclinoid segment (orange) with effective termination in the ophthalmic artery (purple)
ACOM reconsitutes MCA/ACA territory. However, notice that the left M1 segment is deficient, in a Moya-Moya type pattern (yellow). Likely because of this, the normal MCA/ACA watershed is shifted inferiorly (red arrows) and there is signficiant delay in transit at this region. Patients such as this one have a very low tolerance for hypotension.
PCA-MCA convexity collaterals: Left PCA injection shows support of left parietal convexity (red) and essentially the entire temporal lobe (green) via inferior temporal collaterals — a sizeable takeover of MCA territory (PCA typically supplies mesial and variable territories of inferior temporal lobe. Essentially, the PCA-MCA temporal watershed has been shifted to the operculum.
Same view of the left vert injection, in stereo, just for kicks.
Finally, left ECA injection shows that extracranial parietal MMA branch (blue) has been recruited by the brain to produce a synangiosis (brown) whereby the parietal cortex is nourished via pial parasitization of dural branches (MMA in this case)